Day 1 :
Keynote Forum
Mohamed Samir Hefzy
University of Toledo, USA
Keynote: A Biologically Inspired Knee Actuator for a KAFO
Time : 10:05-10:35
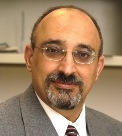
Biography:
Mohamed Samir Hefzy is currently serving as Associate Dean of Graduate Studies and Research Administration of the College of Engineering and Professor of Mechanical, Industrial and Manufacturing Engineering at The University of Toledo (UT), Toledo, Ohio. He has been on the faculty of The UT since 1987. He graduated from Cairo University, Egypt, with a B.E. in Civil Engineering in 1972, and a B.Sc. in Mathematics from Ain-Shams University in 1974. He earned his M.S. in Aerospace Engineering in 1977 and his Ph.D. in Applied Mechanics in 1981, both from The University of Cincinnati. He then received training as a Postdoctoral Research Associate for two years in the Department of Orthopedic Surgery at The University of Cincinnati’s College of Medicine. In December 2003, Dr. Hefzy was elevated to the Grade of American Society of Mechanical Engineers (ASME) Fellow. He is the recipient of many awards, including the 2011 Distinguished Service Award from the ASME.
Abstract:
A person with quadriceps weakness has limited ability to perform knee extension. A knee-ankle-foot orthosis (KAFO) is a common prescription for such disability. Several types of KAFOs are currently available in the market: passive KAFOs, stance-control KAFOs and dynamic KAFOs. In passive KAFOs, the knee joint is kept locked during standing and walking. However the associated uncomfortable walking gait with high energy consumption makes these devices abandoned by patients. Stance control KAFOs block knee motion for weight bearing and allows free rotation in swing phase. However abnormal gait pattern still exists because of the locked knee joint in the stance phase. Dynamic KAFOs are developed to control both stance and swing phases. But those presently available are inconvenient to use and have complex control systems. This research is directed at using superelastic alloys to develop a biologically inspired dynamic knee actuator that can be mounted on a traditional passive KAFO. The actuator stiffness can match that of a normal knee joint during the walking gait cycle. Two superelastic actuators are used for this purpose. They are activated independently. Each actuator is developed by combining a superelastic rod and a rotary spring in series. When neither actuator is engaged, the knee joint is allowed to rotate freely. The stance actuator works only in the stance phase and the swing actuator is active for the swing phase. The conceptual design of the knee actuator was verified using numerical simulation and a prototype is being developed through additive manufacturing for confirming the concept.
Keynote Forum
Michele J Grimm,
Wayne State University, USA
Keynote: The biomechanics of neonatal brachial plexus injury
Time : 10:35-11:05
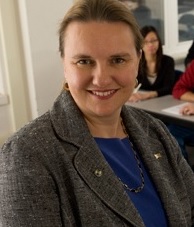
Biography:
Michele J Grimm earned her BS in Biomedical Engineering and Engineering Mechanics from Johns Hopkins and her PhD in Bioengineering from the University of Pennsylvania. She joined Wayne State in 1994 and had the opportunity to work with world leaders in injury biomechanics. In 1997, she began collaborating with an obstetrician on a model of shoulder dystocia and NBPP. She has since become a recognized expert in this area and was the only engineer on the American College of Obstetricians & Gynecologists working group on NBPP. She is a Fellow of ASME and a past chair of the Bioengineering Division of ASME.
Abstract:
Approximately 1 in 1000 infants is noted to have a brachial plexus palsy at the time of birth – resulting in paralysis of the arm. About 10% of these injuries are “permanent” – with residual paralysis after 1 year-of-age. For over 100 years, efforts have been made to understand the mechanism of neonatal brachial plexus palsy (NBPP) and reduce its incidence. Due to the relatively rare nature of the injury, and the sensitivity of studies involving pregnant women and infants, typical experimental methods in injury biomechanics have not been appropriate for NBPP. In the past 15 years, modeling techniques – both computer physical models – have been developed to gain greater insight into NBPP injury mechanisms. But the development of models that cannot be fully validated presents its own challenges. Both computer and physical models have demonstrated that significant stretch of the brachial plexus occurs both as a result of the natural, maternal forces of delivery (uterine contractions and maternal pushing) and any assistive traction applied by the clinician. Available data indicates that stretch due to maternal forces alone is sufficient to cause permanent NBPP in some infants. Currently, there is no way to characterize clinically which individuals will be more susceptible to nerve injury than others. This presentation will review the current state of the art with respect to models of NBPP, with particular focus on the development of computer models, in addition to the current data regarding nerve injury thresholds. The gaps in knowledge that deserve to be addressed will be identified.
Keynote Forum
Lisa A Ferrara
OrthoKinetic Technologies, LLC, USA
Keynote: Innovative biomaterials and surface technologies for medical devices: Observations of mechanotransduction and biologic induction
Time : 11:25-11:55
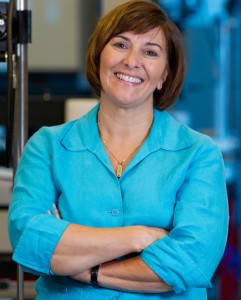
Biography:
Dr. Lisa Ferrara has been faculty at two prestigious academic medical centers and served as the director of the musculoskeletal research facilities. She has received numerous accolades, was involved with the Medical Device Advisory Committee to the FDA, and has provided consulting services about spinal disorders for ABC News. Dr. Ferrara is widely published, provides frequent lectures, serves on multiple scientific and medical advisory boards, and has recently been appointed as a board member to the Advisory Committee for Biotechnology in Southeastern North Carolina.\\r\\n\\r\\nDr. Ferrara previously served as the Director of the Spine Research Laboratory in the Department of Neurosurgery and Orthopedics at The Cleveland Clinic with a research focus on musculoskeletal biomechanics and the development of implantable MEMS sensors for various biomedical applications. She was awarded the Who’s Who Award in Technology in 1999, the NASS Award for Outstanding Research in 1995, and is the recent recipient of the Healthcare Entrepreneur of the Year Award for Coastal North Carolina. \\r\\n
Abstract:
Over multiple decades, orthopedic medical devices have been fabricated from various materials including metals, polymers, ceramics, and allograft tissue. Each material has intrinsic properties that possess certain mechanical characteristics designed for specific anatomical regions and biomechanical parameters. Numerous challenges still exist with the long-term success of many orthopedic devices, yet as innovation continues to progress rapidly, novel materials and surface geometries offer the potential to optimize interface mechanics for improved osseointegration and performance. Therefore, medical devices must be designed with an understanding of the biological and biomechanical principles at the macro, micro, and nano level to offer improved design and implant function. Optimized surface geometries and novel biomaterials have the potential to improve mechanotransduction, while providing greater biological compatibility and biomechanical synergy for long term implantation. These new technologies potentially possess antibiotic/anti-infective properties, micro/nano-surface technologies for improved osseointegration, and controlled surface structures for directed cellular differentiation. Surface geometries and multidimensional load bearing scaffolds have been incorporated into orthopedic implant designs to provide greater contact surface areas at the tissue and bone interface and contribute to improved performance for long term stabilization and immobilization of the tissue. Additionally, the advent of new manufacturing technologies can develop intricate structural scaffolds and nanosurfaces that provide controlled and predictable mechanical stimuli to the cell, where mechanotransduction can be optimized to improve implant function. Therefore, the objective of this work will be to present an overview of a few of these novel technologies, and to present the effects of these novel biomaterials, surface technologies, and structural designs on mechanotransduction and tissue healing.
- Nano-Mechanical Implant Design
Session Introduction
Mark Pitkin
Tufts University School of Medicine, USA
Title: Addressing anisotropy of tissues regeneration in the implants design
Time : 12:00-12:25
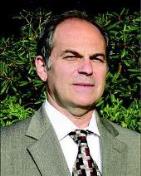
Biography:
Mark Pitkin has completed his PhD in Biomechanics at the Central Institute of Prosthetics Research in Moscow, Russia. He is Professor of Physical Medicine and Rehabilitation at the Tufts University School of Medicine, Boston, MA.
Abstract:
Anisotropy is a fundamental characteristic of tissues regeneration replicating those in the tissues development. If design of an implant is not addressing it properly, the long-term bond between implant and the hosting tissues can-not be sustainable neither in total joint replacement, nor in direct skeletal attachment (DSA) of limb prostheses. Advantages in utilizing anisotropy of regeneration were demonstrated by preferred properties and orientation of the components of the Skin and Bone Integrated Pylon (SBIP) developed by Poly-Orth International, Sharon, MA. Besides optimizing porosity, pores size, particle size and volume fraction, we improved skin-implant and bone-implant interface via preferred orientation of the pylons’ parts. Skin-implant interface: The SBIP is deeply porous perpendicular to the implant’s longitudinal axis and also has perforations in the solid enforcing inserts. Skin cells can therefore penetrate the structure and grow throughout the entire volume of the implant in the “natural” anisotropic direction of regeneration. That creates a skin seal, thus addressing the principal failure modes in existing percutaneous devices: skin regression, marsupialization, permigration, and avulsion. Bone-implant interface: Bone loss after implantation to the marrow canal is observed, and is caused by shield stresses. A variant of the SBIP and a new method of fixation are addressing this problem. Our SBIP-F pylon has side fins that are inserted into precut slots inside the cortical bone walls. The cut out bone activates an Ilizarov type distractional osteogenesis in which the regenerated bone has greater strength than the original bone.
Yoichi Aota
Yokohama Brain and Spine Center, Japan
Title: Entrapment of the cluneal nerves as a forgotten cause of low back pain and leg symptoms
Time : 12:25-12:50
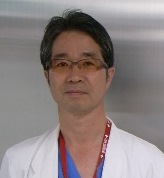
Biography:
Yoichi Aota has completed his PhD from Yokohama City University and Postdoctoral studies from Rush-Presbyterian - St. Luke’s Medical Center, Chicago. He is the Director of department of spine & spinal cord surgery of Yokohama Brain and Spine Center and a visiting Professor of Yokohama City University and Tokyo Medical University. He has published more than 40 papers in reputed journals and has been serving as an Editorial Board Member of repute.
Abstract:
The causes of low back pain (LBP) can be complex and it is often difficult to get an accurate diagnosis. The concept of a relationship between the cluneal nerve and LBP is not new. Following reports by Maigne et al. in 1989 describing that the most medial branch of the superior cluneal nerve (SCN) may become entrapped where the nerve passes through the fascia over the iliac crest, surgeries were undertaken for irritative SCN neuropathy to open the fascial orifice with successful outcomes. Recently, clunealgia has become known as an under-diagnosed cause for chronic LBP or leg pain. We reported that patients with SCN disorders comprised 12% of all patients presenting with a chief complaint of LBP and/or leg symptoms in our clinic and approximately 50% of SCN disorder patients had leg pain and/or tingling. There are several anatomical variations in the running courses of the SCN. On the other hand, entrapment of the middle cluneal nerve (MCN) within the long posterior sacroiliac ligament (LPSL) has been sporadically suggested as a potential cause of LBP and peripartum pelvic pain. In my experience, SCN entrapment is often associated with MCN entrapment. MCN entrapment under the LPSL is a potentially under-diagnosed cause of chronic LBP. Knowledge of this clinical entity would avoid unnecessary spinal surgeries and sacroiliac joint fusion. SCN and/or MCN blocks are useful not only for obtaining pain relief, but also to confirm the diagnosis by pain relief after injection.
Boris I. Prilutsky
Georgia Institute of Technology
USA
Title: Adaptation of bone-anchored limb prosthesis for locomotor behaviors
Time : 12:50-13:15
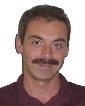
Biography:
Boris I Prilutsky has received his PhD from Latvian Research Institute of Traumatology and Orthopedics, Riga, former USSR. He is the director of Biomechanics & Motor Control laboratory in the School of Applied Physiology at Georgia Tech, Atlanta, USA. He has published 2 books and over 60 scientific papers, has been serving as a review panel member of National Institutes of Health & National Science Foundation, USA and Natural Sciences and Engineering Research Council, Canada, and has beenan editorial board member of Scientific Reports, UK.
Abstract:
Bone-anchored limb prostheses have been utilized in Europe for over 20 years. Although this technology has many advantages over traditional socket prosthesis attachments, it has not been approved for use in US due to high risk of skin infection at the skin-implant interface. The recently developed porous titanium pylon for skin and bone integration (SBIP, Poly-Orth International, USA; Pitkin, Raykhtsaum, 2012) has demonstrated the potential for skin-bone ingrowth into the pylonthus reducing or preventing infections. Our laboratory has utilized animal models (rodents, felines) to investigate integration of SBIP pylons with residual limb after the implant is loaded during every-day prosthetic walking and standing. The animals wear a trans-tibial J-shape prosthesis over several months.Level and slope prosthetic walking is recorded using 3D motion capture and force plates. Biomechanical analysis is used to determine contributions of the prosthetic and contralateral hindlimb to joint moment, power and work production. At the end of study, animals are euthanized using deep anesthesia andlimb with implant is harvested for histological analysis. Our current results have demonstrated that animals adopt bone-anchored prostheses for standing and locomotion over several months. Although load on prostheses is reduced by approximately 30-40%, it appears caused by loss of the ankle joint, important source of mechanical energy for locomotion. Histology shows substantial skin-bone ingrowth into implant. The results strongly suggest that the SBIP can successfully be used in bone-anchored prosthesis and has the potential for reducing skin infection.
- Clinical Biomechanics
Session Introduction
Muhammed Wasif Rashid Chaudhary
Ahalia Hospital, United Arab Emirates
Title: Basics of biomechanics of the neuromusculoskeletal system and research opportunities
Time : 14:00-14:25
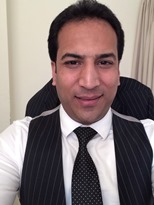
Biography:
Muhammed Wasif Rashid Chaudhary, MBBS, MBA, CSSGB, CTQM, CPHQ is working as Asst. Medical Director in United Arab Emirates. He has 15 years of experience in healthcare field. He had worked also as Medical Superintendent and Quality Manager 8 years in the same health care facility. His interest is in continuous quality improvement and patient safety. He is licensed general practitioner and continuing his responsibility as a physician. He is certified professional in healthcare quality (CPHQ). That credential covers field of quality, case/care/disease/utilization and risk management and emphasizes how all these programs and processes integrate into an effective system
Abstract:
Biomechanics is the study of mechanics (loads, motion, stress, and strain of solids and fluids) applied to biological systems. Musculoskeletal Biomechanics specifically focuses these methods for studies of the musculoskeletal system. This includes studies of the form and function of tissues including bone, cartilage, ligament, tendon, muscle, and nerve, at multiple scales ranging from the single cell to whole body. My presentation will provide a general overview of the biomechanical principles associated with the neuromusculoskeletal system. In particular I will review, the structure and properties of the neuromusculoskeletal system, and show how the various components of this system can be idealized and described in mathematical terms. The presentation begins with an overview of the mechanical properties of muscle, tendon, ligament, and cartilage, finishing off with a review of the ongoing research opportunities in Biomechanics, including a brief look at musculoskeletal tissue engineering, ergonomics, musculoskeletal adaptation, and tissue mechanics.
Mohamed Hamdy Doweidar
University of Zaragoza
Spain
Title: A 3D numerical model of cell differentiation and proliferation
Time : 14:25-14:50

Biography:
Mohamed Hamdy Doweidar is a Full Associate Professor at the Mechanical Engineering Department, University of Zaragoza, Spain. Besides, he is a Member of the Group of Structural Mechanics and Materials Modeling (GEMM), the Biomedical Research Networking Center in Bioengineering, Biomaterials, and Nanomedicine (CIBER-BBN), the Aragón Institute of Engineering Research (I3A), and the European Society of Biomechanics (ESB). He has participated in many national and international investigation projects. He has authored and co-authored many books and numerous articles in international journals. His investigation interests include Computational Biomechanics, Cell Simulation, Finite Element Method, Natural Element Method and Computational Fluid Dynamics.
Abstract:
Mesenchymal stem cells (MSCs) have the ability to differentiate into many cell phenotypes such as fibroblasts, chondrocytes, osteoblasts and neuronal precursors. Experimental studies have demonstrated that mechanical characteristics of the substrate, such as substrate stiffness, fluid flow and mechanical forces can govern their fate even in absence of biochemical factors. However, the signaling mechanism behind this process is not well understood. The main objective of the present work is to develop a numerical model to study the influence of substrate mechanical conditions on cell differentiation, proliferation and apoptosis. To formulate the model, a discrete finite element approach has been appreciated. For the sake of simplification, it is assumed that the cells have a spherical shape; however it is possible to consider any other cell configuration. We consider that these processes are related to the received mechanical signals by cells and its maturation time. It is assumed that the cell fate is governed by the cell internal deformation experimented during cell migration. Consistent with experimental observations, our findings indicate that within soft (0.1-1 kPa), intermediate (20-25 kPa) and hard (30-40 kPa) substrates MSCs proliferate and then differentiate into neuroblasts, chondrocytes and osteoblasts, respectively. The traction force generated by a specific cell phenotype can increase (osteoblasts and chondrocytes) or decrease (neuroblast) during differentiation. In contrast, in all cases the proliferation of a typical cell considerably increases the average cell traction force due to the cell-cell interaction. Hence, greater the substrate stiffness, the higher is the cell differentiation and proliferation rate.
Udi Sarig
Nanyang Technological University, Singapore
Title: Biophysical characterization of the recellularization effect: Restoring functional properties of ECM based constructs towared the native state
Time : 14:50-15:15
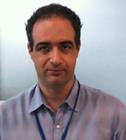
Biography:
Udi Sarig has completed his PhD from the Technion – Israel Institute of Technology (IIT) and is currently a Postdoctoral fellow at the Nanyang Technological University (NTU) in Singapore. He is a co-author of more than 20 publications in international recognized peer reviewed journals and scientific conferences, and is currently serving as a group leader in the Singapore Technion Alliance for Research and Technology (START), under the Campus for Research Excellence and Technological Enterprise (CREATE) program: The regenerative medicine initiative in cardiac restoration therapy.
Abstract:
‘Functional tissue engineering’ (FTE) – a tissue engineering (TE) subfield – employs various cellularized biomaterial scaffolds for the “engineering of load bearing tissuesâ€. To generate more biomimetic materials, various extracellular matrix (ECM) scaffolds were isolated through decellularization. However, decellularization represents a trade-off between excessive ECM damage and preservation of ECM ultrastructure and bioactivity. Indeed, vast research has identified biophysical effects of the ECM on cell survival, proliferation, migration, organization, differentiation and maturation, with clear implications for FTE. Surprisingly though, no study to date, to the best of our knowledge, provided clear methods and understanding on the reciprocal effects of cellularization on the cellularized ECM scaffolds biophysical properties, under physiological-like conditions. We hypothesized that by re-cellularizing porcine ventricular ECM (pvECM, serving as a model scaffold) some of the original myocardial tissue biophysical properties can be restored, concerning scaffolds surface and bulk modifications consequent to cellularization. We therefore performed a systematic biophysical assessment of pcECM scaffolds seeded with human mesenchymal stem cells, a common multipotent cell source in cardiac regenerative medicine. The results obtained were compared to acellular pcECM and native ventricular tissue serving as negative and positive controls, respectively. We report a new type of FTE study in which cell interactions with a composite-scaffold were evaluated from the perspective of their contribution to the construct surface (FTIR, WET-SEM) and bulk (DSC, TGA, uni-and bi-axial mechanical testing) biophysical properties. Such an approach yields important methodologies, understanding, and data serving both as a reference as well as possible ‘design criteria’ for future studies in FTE.
Yoichi Aota
Yokohama Brain and Spine Center, Japan
Title: Workshop: Entrapment of superior and middle cluneal nerves as an unknown cause of low back pain and pseudosciatica
Time : 15:35-17:10
Biography:
Yoichi Aota has completed his PhD from Yokohama City University and Postdoctoral studies from Rush-Presbyterian - St. Luke’s Medical Center, Chicago. He is the Director of department of spine & spinal cord surgery of Yokohama Brain and Spine Center and a visiting Professor of Yokohama City University and Tokyo Medical University. He has published more than 40 papers in reputed journals and has been serving as an Editorial Board Member of repute.
Abstract:
Low back pain (LBP) is one of the most common problems that most people suffer at some point in their life. There are many sources of LBP. In most LBP patients, the exact cause of LBP is not clear. Thus, one of the most difficult task with LBP is to identify the actual pain generator. Large epidemiological studies show that 20% to 37% of patients with back pain suffer from a neuropathic pain component. superior and middle cluneal nerves (SCN / MCN) entrapment must not be forgotten as cause of neuropathic LBP. Although many chiropractists, physiotherapists, and archipuncture seem to know this etiology, so far unfortunately, it is not widely recognized in orthopaedic or neurosurgeons. SCN and MCN supply the skin overlying the posteromedial area of the buttock. Previous studies illustrated that the SCN is derived from the cutaneous branches of the dorsal rami of T11-L5. In spite of more than 50 years of surgical experiences in clunealgia, information of clunealgia is limited. Entrapment of SCN/ MCN induces low back pain and leg symptoms. SCN entrapment occurs where SCNs pierce fascial attachment at posterior iliac crest. Although this clinical entity had been known as a rare cause of unilateral low back and/or buttock pain, recently, clunealgia has become known as an under-diagnosed cause for chronic LBP or leg pain. In a recent prospective study, Kuniya et al. reported that patients with SCN disorders comprised 12% of all patients presenting with a chief complaint of LBP and/or leg symptoms in their clinic and approximately 50% of SCN disorder patients had leg pain and/or tingling. The MCNs can become spontaneously entrapped where this nerve pass under the long posterior sacroiliac ligament. Clunealgia is underdiagnosed and should be considered as a potential cause of severe low-back and/or leg symptoms. The symptoms of clunealgia can be very severe and mimicked a radiculopathy and disc disorders in lumbosacral spine. Clinicians should be aware of this clinical entity and avoid unnecessary spinal surgeries and sacroiliac fusion. Techniques in SCN surgeries may differ from those in other common peripheral nerve surgeries because branches of SCN/MCN are thin and requires release in multiple branches. This workshop is to draw attention by pain clinicians in SCN/ MCN entrapment by comprehensively reviewing its historical perspective, anatomical background, clinical symptoms with respect of differential diagnosis and surgical tips.
- Biomechanical Materials and Implant Designs
- Biomechanics of the Human Neuromusculoskeletal System
- Biomechanical Devices
Session Introduction
Lisa A. Ferrara
OrthoKinetic Technologies, LLC
Southport, North Carolina
Title: Titanium Truss Structures as Conduits for Bone Healing Through Multiplanar Stress Distribution
Time : 10:00-10:25
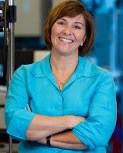
Biography:
Abstract:
Introduction: Truss designs are composed of triangular units connected at nodes to provide multiple planes of structural stability. The triangle will not change shape when the lengths of the sides are fixed, resulting in ability to resist significant axial forces. Forces and reactions to them are considered to act only at the nodes and result in forces in the members which are either tensile or compressive, where bending or rotational moments are explicitly excluded, thus bending or rotational moments are expressed as stress transfer to surrounding substrate located at the truss interfaces. This function makes the truss a viable structure for orthopedic devices that are constantly exposed to bending moments, where the greatest moments can occur at the terminal endpoints of devices. A three-dimensional titanium truss structure was fabricated through additive manufacturing techniques to create a multidimensional load bearing scaffold for structural support and fusion incorporation in orthopedic applications. The aim of this study was to develop a series of titanium scaffolds composed of multiplanar truss structures to assess structural integrity and stress distribution for orthopedic use. A comprehensive battery of mechanical tests and validated finite element models [FEA] were performed to determine the load bearing strength and endurance characteristics, as well as the stress profiles distributed throughout the truss structures under multiplanar loading. Methods: Static and dynamic fatigue tests of multiple truss structures with different strut diameters were conducted to measure the yield and ultimate strength and fatigue endurance load limits for multiple planes of physiological motion. FEA models were constructed and loading on each structure mimicked the actual mechanical testing, followed by a validation of each model with the actual test results. Once the models were validated, they were loaded in multiple planes to provide a profile of stress distribution throughout the truss structures. Results: Mechanical results of the truss structures exhibited yield and ultimate strength, stiffness, and fatigue endurance at loads of at least 10 to 15 times stronger than physiological loads in all planes of loading. The open architecture of the truss structure counteracted the high global stiffness measured by distributing stresses across a much greater surface area in multiaxial planes than that of traditional titanium implants, thus reducing the potential for stress risers at the bone interface. The FEA demonstrated stress transfer to truss units deep within the implant, as well as on the surfaces, for all planes of physiological loading. Conclusions: This study found the strength of the truss structure combined with its open architecture created an ideal scaffold that distributed multiplanar forces (stress) throughout the truss formations to all interfaces of the bone surrounding each strut. The end result is a mechanically sound structure with improved stress distribution to the superficial and deeper truss structures. This increased stress transfer is non-destructive to its mechanical integrity and will theoretically result in greater transfer of microstrain to the bone it contacts. Although not the topic of this abstract, an early in vivo animal study assessing bone fusion through these truss structures in sheep intervertebral discs found structurally sound remodeled bone at early time periods. These results potentially support this theory of stress-strain transfer to individual struts within truss structures, which lead to potentially earlier bone incorporation which may promote earlier global stabilization across a bone defect, resulting in augmented bone recruitment.
Abigail Jaitman
University of Warwick, UK
Title: Multi-segment musculoskeletal foot model
Time : 10:25-10:50
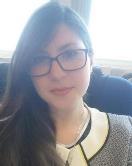
Biography:
Abigail Jaitman is a last year Doctoral candidate at Warwick University, UK. Her main area of research is Biomechanics and how it is affected by diabetes; using electromyography, motion capture, plantar pressure and image processing to support state space models. She holds a 6-year BSc in Electronic Engineering from University of Belgrano, Argentina. Since 2009 she has been doing research on Dosimetry (Medical equipment CONICET – UB), and since 2011 is Research Assistant for Dr. Rojas (RSNA & ESR) working on the Mammographic field. She presented at conferences: ECR 2014, PGBiomed/ISC 2014, XIX ICMMB, ECR 2015 and recently published in JMMB.
Abstract:
The difficulties arising in foot modeling are inherent in its complex composition. Most models simplify the foot geometry, structure, materials and kinetic analysis. To overcome this challenge, in this study a new approach is presented, combining gait analysis, plantar pressure and image processing together with biomechanical principles to develop a multi-segment foot model. The model consists of four segments (Phalanges, Midfoot+Metatarsals, Calcaneus, Talus) to represent the foot according to its function, and a fifth segment for the rest of the body. In order to support the model in terms of geometry and parameterization, 10 series of healthy feet images (X-rays, CT and MRI provided by UHCW consultants) were analyzed. Arch height was calculated from motion capture experiments (10 subjects) and the ground reaction force distribution throughout the gait cycle was obtained through plantar pressure analysis on different subjects (14), all experiments complying with data protection requirements. The plantar soft tissue is modeled as system parallel spring dampers. The model considers four relevant joints: Metatarsophalangeal, Transversetarsal, Talocalcaneal and Taloclural. Regarding muscle activation, 13 muscles (both extrinsic and intrinsic) are included using modified Hill muscle model, composed by a contractile element, spring and damper in parallel, to model the muscle, in series with another spring, the latter to model the free tendon. In order to find a set of fitted parameter values for the stiffness and damping coefficients, parameter estimation and optimization is applied on per-subject basis, yielding a minimum absolute error between the measured and simulated trajectories for the model’s joints.
Natacha Rosa
University of Porto
Portugal
Title: Nailing stability during tibia fracture early healing process: A biomechanical study
Time : 11:10-11:35
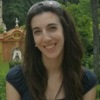
Biography:
Natacha Rosa worked as a Researcher in Faculty of Engineering, University of Porto between September 2011 – December 2012. Currently she is working as PhD grant holder (FCT) Faculty of Engineering, University of Porto
Abstract:
Intramedullary nailing is one of the oldest types of surgical fracture treatment and is now considered a standard procedure for the surgical management in most tibia diaphysis fractures treatment. Although bone has a unique capability to repair following trauma, it is well accepted that the mechanical conditions at the fracture site influence the healing outcome. The inter fragmentary motions are greatly determined by the stability of the bone-implant assembly and also the nature and magnitude of the loads applied to the limb. This study consists in a biomechanical evaluation of the stability of a tibia-intra medullary nail construction model and the amount of inter fragmentary movements, as closely as possible to the physiological loading conditions for partial-weight bearing which may occurs during a patient’s early recovery stage. To avoid inconsistency of cadaveric bone samples, a synthetic bone model was used in the independent load cases experiments to determine the three-dimensional stability of the assembly. This study allowed a better understanding about intra medullary nail fixation devices configuration, the amount of inter fragmentary motion that occurs during patients early healing phase, and will help develop future strategies to improve intra medullary nail implants for a more favorable mechanical healing environment to occur.
Fatima Abu Baker Hamad
University of Gezira, Sudan
Title: Occupational and prostate cancer risk
Time : 11:35-12:00
Biography:
Fatima Abu Baker Hamad is a PhD student in Molecular Biology, Institute of Endemic Diseases, at the University of Khartoum (2012-2016), an MS in Biochemistry & Nutrition from Gezira University, and a BS in Biochemistry & Nutrition from Khartoum University. She is particularly a member in Sudanese Cancer Research Group, Sudanese Environment conservation Society-Wad Madani Branch and member in RAMA Society for cancer patient’s aid- National Cancer Institute (NCI), Gezira University. She published and presented different scientific papers in more than 10 conferences all over the world. She is focused on agriculture communities, chemical contamination, occupations, environments and cancer risk factors.
Abstract:
The rapidly increasing incidence of prostate cancer in Sudan calls for attention to the etiologic and prevention of this type of cancer in old men. Potential risk factors that are mentioned in Sudanese prostate cancer patients are age, education level, unhealthy habits, the body mass index and occupation. A population based case–control study recruited 237 men with a diagnosis of confirmed prostate Cancer and 237 controls randomly selected from the community. Thirteen occupations and 8 industries were selected for analyses to estimate the odds ratio between each occupational circumstance and prostate cancer with control for potential confounders. History of farmer was associated with a highly significant increased the risk for prostate cancer OR (3.711; 95% CI, 2.722-5.058), as was exposure to pesticides was associated with a highly significant increased OR (3.512; 95% CI, 2.611-4.725, P<0.000). Agriculture industry were strongly significantly elevated the risk for prostate cancer, as well as miscellaneous services wasn’t associated but it had significant affected OR (3.439 and 0.506; respectively, P<0.000). Farmer and Horticulturalists, mixable workers and Businessmen were relatively high odds ratios; also these were high statistically significant (P<0.000). These results suggest positive associated was appeared between some occupations, industries and increased the risk for prostate cancer in Sudan. Furthermore it needs more attention to preventing and curing the agriculture community.
Tejpal Singh
North West Group Of Institutions Dhudike, Moga, India
Title: A comparative study of various surface finishing techniques for parts produced by fused deposition modelling
Time : 12:00-12:25
Biography:
Tejpal Singh is from the North West Group Of Institutions Dhudike, Moga, India. His research interests are Surface roughness, Wax coating and Dimensional accuracy
Abstract:
The objective of the present study is to experimentally reduce the surface roughness of plastic parts by four different post processing techniques such as wax coating, mass finishing, chemical treatment, and sand paper to evaluate the most suitable one. The experiments have been conducted on ABSplus material which is a most popular material for FDM machine. Surface roughness and Dimensional accuracy were selected as the output responses for the present investigation. After the surface finishing, the parts were used as patterns in investment casting process to compare the value of surface roughness and dimensional accuracy. The best method for minimum surface roughness was chemical treatment.
Dr. Behrooz Sepehri
Islamic Azad University, Iran
Title: On the effects of a FGM plate on stress pattern at fractured site
Time : 13:10-13:35
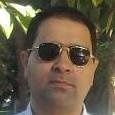
Biography:
Behrooz Sepehri completed his PhD at the age of 32 years in Medical Engineering- Biomechanics in Science and Research Center Branch, Islamic Azad University, Tehran, Iran. He is working as Lecturer for courses related to areas of Biomechanics, such as Introduction to Biomechanics, Biomechanics of Bone Diseases, and Artificial Organs and Limbs. He has also published some 15 papers in related areas
Abstract:
Orthopedic plates are currently used in bone healing process. However they cause density loss in underlying bone because of the change in natural stress patterns. The aim of this study was to evaluate a newly developed bone plate using functional graded material, FGM in term of stress pattern. In the present study, 3D finite element models of tibial bone plate with variable stiffness of a graded material and traditional bone plates made of stainless steel and Ti alloy have been developed by using the ABAQUS software. Effects on the predicted stresses at the fracture site in the presence of a distance between the plate and fractured bone were also studied. For this purpose, a 3D model of tibia was created with the exact geometry of the real bone geometry by using CT scan images of a human left leg. Results showed that the bone plate with graded material offers less stress shielding to the bone, providing a higher compressive stress at bone to induce accelerated healing in comparison with Ti alloy and stainless-steel bone plate. Results also showed that the use of non-contact plates provide a favorable mechanical environment for the following fracture healing.
- Implant/Transplant Surgeries
- Biomedical engineering
Session Introduction
Ash Harkara
VOLMO Ltd
UK
Title: Optimisation of implant position in cemented femur for lowest value of micromotion
Time : 11:20-11:45

Biography:
Ash Harkara is a founder and Managing Director of VOLMO. Ash is a scientist entrepreneur specializing in building culture of innovation and collaboration that drives growth, profitability and creates value. His talent for developing global visions and strategies, translating them into executable plans, a fierce commitment to customer intimacy, operational excellence and a passion for empowering people has helped Ash successfully shape and steer organizations towards growth and shareholder value creation. A decisive, intelligent risk taker, he challenges paradigms by ‘doing the right things rather than doing things right’. Ash has MBA and 20 years experience in academics and industry across various roles and functions. Having worked with clients across all the continents has equipped him with transnational business acumen needed to successfully manage global businesses, multimillion dollar budgets and P&L to ensure profitability and growth. By helping businesses get the basics right and take a leaner approach, he enables them to adapt to changing environments and execute on strategies that deliver results and create share holder value. With a PhD in computational modeling, Ash has a keen interest in patient specific device development using 3D medical image processing, finite element analysis/computational fluid dynamics and 3D printing.
Abstract:
Total hip replacement (THR) is one of the most common and effective surgical procedures performed worldwide with the purpose of improving the quality of life of patients suffering from hip disorders. There are two major types of artificial hip replacements: cemented and uncemented. This paper presents finite element approach to measure micromotion along the stem/cement and cement/bone interface. A response surface model (RSM) was created and used to determine the best implant position for lowest value of micromotion. In this study finite element model of femur was created from 3D CT scan data using ImageSim software from VOLMO. Surface model of femur in STL format was exported into ImageSim's CAD environment where femur resection, implant positioning and alignment were done. The final assembled model of bone, cement and implant was used as the base model for creating new models. Java scripting functionality within the tool provided a robust environment for automatically rotating the implant to a new angle, exporting STL, volume meshing, applying load boundary condition and exporting volume mesh. This technique provided an efficient approach for generating new models with different implant positions in the femur bone. Around 25 models were generated in less than twelve hours. These models were then simulated in DYNAMIS solver available internally in the environment.
Emre KARADENIZ
Umraniye Training and Education Hospital, Istanbul, Turkey
Title: A New Fixation Device and Technique: EFECE
Time : 11:45-12:10
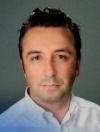
Biography:
Emre Karadeniz has completed his MD at the age of 24 years from Osmangazi University School of Medicine and residency from Baskent University School of Medicine. He has completed his spine surgery fellowship from Hacettepe University and Istanbul Spine Center. He is the lecturer of Umraniye Training and Research Hospital. He is the inventor of EFECE fixation systems. And he has more than 10 national and international patents.
Abstract:
EFECE is a patented fixation device. It is cylinder shaped and contains 2 pieces that catch each other with threads. EFECE, contains 3 balls in cone shaped gloves.(Video: https://www.youtube.com/watch?v=AloRcFcae6g) It has surgical tool set which is a new technique. With EFECE systems; along the EFECEwires, surgeon may compress the fracture line and after locking EFECE, fixation may be achieved. The smallest EFECE, which is with 6mm radius, can be used with 1.2mm EFECE wire. 6mm EFECE resist up to 73kg of distraction force. EFECE scales are; from 6mm to 20mm radius. EFECE wire scales are; from 1.2mm to 3mm.
Technique: After reducing the fracture, EFECE wire should be passed across the fracture line. With percutaneous technique, EFECE should be advanced on the EFECE wire to the bone cortex, with the help of the patented tools. Then EFECE should be fastened. From the counter side of the EFECE wire, the second EFECE should be advanced to the bone cortex. Then, EFECE wire should be tensioned with EFECE wire stretcher and the EFECE should be fastened. The remaining part of the EFECE wire should be cut with the EFECE wire cutter. Implant removal is with the help of EFECE magnets.
EFECE systems are able to achieve fixation with the help of thin EFECEwires. The fixation strength is not related to bone quality. Thin EFECEwire achieve fixation in difficult bone anatomy like elbow. Technique is completely percutaneous. Indication scale is wide. Implant removal with magnets is also a new approach for implant technologies.
Lisa A Ferrara* and Diane Dobrea#
*OrthoKinetic Technologies, USA
# Kapstone Medical, USA
Title: Workshop: Designing and evaluating protective gear and implants for safety and effectiveness
Time : 12:10-13:10- Break-13:55- 15:55
Biography:
Dr. Lisa Ferrara is currently the Owner and President of OrthoKinetic Technologies LLC, and OrthoKinetic Testing Technologies, LLC, a medical device consulting and certified test facility that provide a turn-key approach for medical device evaluation. Academically, she was on staff for the Departments of Neurosurgery and Orthopedic Surgery respectively, at The Cleveland Clinic Foundation (CCF) in Ohio, where she founded the Spine Research Laboratory and served as the director from 1999 until 2003. During her 25 years of academic research, she has authored more than 50 technical publications, 25 book chapters, has been granted two patents, and given over 50 invited presentations on novel technologies, biomaterials, nanotechnology and nanomedicine, musculoskeletal and spinal biomechanics, surgical stabilization techniques and medical devices. She also serves on numerous scientific advisory boards and was the recent recipient of the Healthcare Entrepreneur of the Year Award for Coastal North Carolina, was highlighted as an industry expert in medical device testing and analysis, and was named Woman of the Year in 2015 by the National Association of Professional Women.
Abstract:
Innovation starts with a novel idea that has the potential to evolve into a viable product which can address a specific market need. The criteria for successful product development are multifaceted and involve a multidisciplinary team to ensure that the product behaves in a safe and effective manner. Often, the greatest challenges the team may face are during the early phases of product development. Entry into the product lifecycle of a new technology involves the coordination of multiple resources and experienced experts with diverse backgrounds that can provide guidance on the market need, patent landscape, verification and validation protocols, manufacturing capabilities, potential risks and mitigation. Additionally, there are a number of specific processes outlined in the product life cycle that provide the pathway for successful product development from the early concept stage to final commercialization. Thus, ensuring that a safe product, which functions per its intended use is brought to market, involves many iterative processes throughout the product lifecycle. Therefore, the objective of this workshop will be to provide an overview of product development from early concept to commercialization, which will include a discussion of the potential risks and pitfalls that are commonly faced during the many phases of development.
Poster Presentations 15:55-16:55